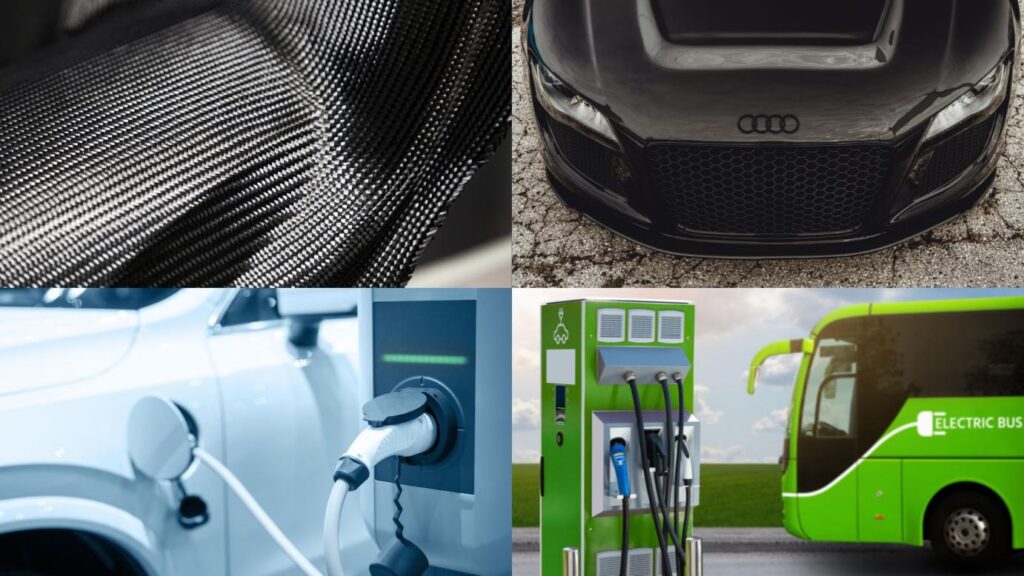
With over 10 years of experience researching and developing advanced composite materials for the automotive industry, I have an in-depth understanding of the capabilities and limitations of these materials. As an expert in this field, I aim to provide comprehensive and accurate information to help engineers, designers, and manufacturers make informed decisions about selecting and working with composites.
Lightweighting the Automotive Industry
The automotive industry is actively seeking ways to reduce vehicle weight to meet emissions regulations and improve fuel efficiency. Replacing heavy steel components with lightweight composite materials is a proven weight reduction strategy. Composite materials offer high strength-to-weight ratios, corrosion resistance, and design flexibility.
What are Composite Materials?
A composite material consists of two or more constituent materials with significantly different physical or chemical properties. When combined, they create a new material with enhanced overall properties. The two main categories of composites are:
- Polymer matrix composites (PMCs): Consist of a polymer resin reinforced with fibers. The resin (such as epoxy or polyester) acts as the matrix to bind and transfer loads to the fibers.
- Metal matrix composites (MMCs): Consist of a metal matrix reinforced with ceramic or metallic fibers. Common metal matrices include aluminum, magnesium, and titanium.
Benefits of Using Composites in Automotive Manufacturing
Composites offer several advantages over traditional automotive materials: Lightweighting: Composites have high strength-to-weight ratios compared to metals, allowing for weight reductions of 40-60% in some components. This directly reduces fuel consumption. Design flexibility: Complex shapes and integrated functions can be molded in a single step, enabling innovative styling and part consolidation. Corrosion resistance: Composites withstand harsh operating environments, including moisture, chemicals, and road salts. This improves durability and longevity. Part performance: Tailoring the fiber type, orientation, and resin system allows engineers to design parts with precise mechanical characteristics. Processing efficiency: Near-net-shape molding minimizes machining and finishing steps. The smooth surfaces of composites also reduce assembly effort. Sustainability: Natural fibers and some resin systems use renewable resources and enable closed-loop recycling processes.
Current and Future Use of Composites in Automotive Manufacturing
Composites usage in mass production vehicles is steadily increasing as manufacturing processes evolve to enable high volumes at low costs. Applications seeing rapid adoption include:
- Structural components: Replacing steel in frames, bumpers, body panels
- Powertrain components: Reducing weight in engine blocks, transmission cases, drive shafts
- Interior components: Door panels, seat structures, instrument panels
- Exterior attachments: Mirror housings, spoilers
Luxury vehicles and high performance sports cars often showcase innovative uses of advanced composites. These showcase applications demonstrate viability and spur wider adoption as costs decrease. Industry experts predict composites usage in automobiles will grow from a $4.3 billion market in 2020 to over $10 billion by 2030.
Composite Materials Manufacturing Processes
There are several common manufacturing processes used to fabricate composite components for automotive applications:
Open Mold Processes
Hand Layup: Reinforcing fibers are placed manually in an open mold and saturated with resin by pouring, brushing, rolling, or spraying. Low cost, but labor intensive. Spray-up: Simultaneously sprays reinforcement and resin into an open mold. Very fast, low cost process with limited performance. Filament Winding: Fibers are impregnated with resin, then continuously wound over a rotating mandrel to build hollow structures. Used for pipes, drive shafts, compressed gas tanks.
Closed Mold Processes
Compression Molding: Sheet molding compound (SMC) or bulk molding compound (BMC) are placed in mold, then compressed and cured with heat. High volume process for complex parts. Resin Transfer Molding (RTM): Dry reinforcement preform is placed in mold, then resin is injected under pressure and cured. More precise control than open mold processes. Pultrusion: Continuous fibers are pulled through a resin bath, then shaped and cured in a heated die. Makes constant cross-section parts like rods and beams. Vacuum Infusion: Fibers are laid dry into mold, then resin flows into mold under a vacuum. Achieves very high fiber volumes and precision.
Emerging Processes
Automated fiber placement, tailored fiber placement, and advanced molding processes aim to reduce cycle times and improve quality and repeatability.
Process | Description | Typical Applications |
---|---|---|
Automated Fiber Placement (AFP) | Robotic head adds bands of pre-impregnated tows with heat and pressure | Large, contoured aerospace structures |
Tailored Fiber Placement (TFP) | CNC filament winding that varies tow width and angle | Pressure vessels, golf club shafts |
High Pressure RTM (HP-RTM) | RTM process with injection at higher pressures | Structural components with deep ribs and bosses |
Compression RTM (C-RTM) | Combines compression and RTM for faster cycles | High volume automotive parts |
Optimizing Composite Materials Selection
With the wide range of fiber and resin options for polymer composites, selecting the right materials for an application can be challenging. Here are key considerations when specifying materials: Loading conditions: Will the component experience high static loads or cyclic fatigue loading? Tensile, compressive, flexural, impact? Understanding load types and magnitudes will guide fiber, weave, and resin selection. Environment: What temperatures, exposure to moisture and chemicals, and potential damage threats will the component experience in service? Materials must retain adequate properties in the operating environment. Performance requirements: Which properties are critical? High strength, stiffness, durability, damage tolerance, creep resistance, thermal stability? Different fibers and resins enhance different properties. Manufacturability: Can the selected materials and design enable high volume production at the targeted cost? Process characteristics like cycle time, tooling cost, scrap rate impact manufacturing efficiency. Collaborating with a composites design expert early in the component development process is highly recommended to ensure all design and manufacturing requirements are met.
The Composite Materials Future
The unique performance benefits of composite materials make them an essential part of building lighter, more environmentally friendly vehicles. As manufacturing processes mature to enable cost-effective mass production of structural composite components, usage rates will continue to grow rapidly. Industry commitment to research and development is also accelerating composite material innovation – stronger fibers, snap-curing resins, and advanced recycling processes open expansive possibilities for next-generation vehicles. Lightweighting with composites is transforming automotive design and manufacturing. By leveraging the expertise of specialists in selecting high-performance composites matched with efficient manufacturing processes, engineers can fully capitalize on the promise of composites for transportation applications.